Xeroderma Pigmentosum, Complementation Group A
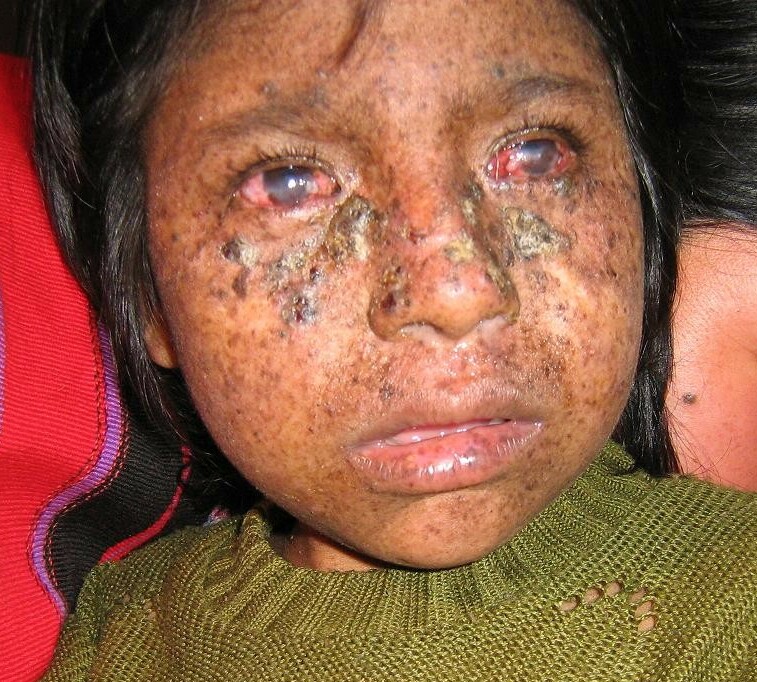
A number sign (#) is used with this entry because xeroderma pigmentosum complementation group A (XPA) is caused by homozygous or compound heterozygous mutation in the XPA gene (611153) on chromosome 9q22.
DescriptionXeroderma pigmentosum is a genetically heterogeneous autosomal recessive disorder characterized by increased sensitivity to sunlight with the development of carcinomas at an early age. Some patients develop neurologic symptoms or a more severe clinical phenotype known as de Sanctis-Cacchione syndrome (278800) (Satokata et al., 1992).
See also XPB (610651), XPC (278720), XPD (278730), XPE (278740), XPF (278760), XPG (278780), and variant XP (XPV; 278750).
Clinical FeaturesRuder (cited by Cockayne, 1933) observed the condition in 7 out of 13 sibs.
Kanda et al. (1990) indicated that most patients with group A XP, the most common form in Japan, show involvement of the central and peripheral nervous systems in addition to cutaneous lesions. The authors detailed the pathologic changes in the peripheral nervous system.
Bootsma and Hoeijmakers (1991) compared the clinical and biologic characteristics of 7 excision repair-deficient XP complementation groups. They found that XPA and XPC have high relative frequency; XPD and XPF have intermediate frequency, and the other 3 are rare. For each of the 7 types, they tabulated the occurrence of clinical manifestations in 4 areas, skin cancer, neurologic abnormality, symptoms of Cockayne syndrome (see CSA, 216400), and trichothiodystrophy (601675), as well as the levels of unscheduled DNA synthesis after UV exposure and of UV sensitivity.
Salob et al. (1992) reported a Pakistani girl, the offspring of second cousins, who developed photosensitivity at the age of 2 years; at the age of 9 years, she was found to have typical xeroderma pigmentosum together with bone marrow hypoplasia of a type seen in Fanconi anemia (FA; 227650). Salob et al. (1992) suggested that this was not a chance association but that the bone marrow hypoplasia reflected an increased susceptibility of the patient's hematopoietic stem cells to non-UV mutagens.
Kraemer et al. (1994) examined reports of 132 patients with xeroderma pigmentosum. Malignant skin neoplasms were present in 70% of the patients with XP at a median age of 8 years (50 years earlier than in the U.S. white population); 57% of the patients had basal cell or squamous cell carcinoma, and 22% had melanoma. The frequency of melanomas, like the frequency of nonmelanoma skin cancers, anterior eye cancers, and tongue cancers, but unlike that of internal neoplasms, was increased 1,000-fold or more in patients with XP who were younger than 20 years. As in the general population, the anatomic distribution of melanomas was different from that of nonmelanomas in the patients with XP. Data were interpreted as suggesting that DNA repair plays a major role in the prevention of cutaneous cancers in the general population, and that sunlight exposure is responsible for the induction of melanoma as well as nonmelanoma skin cancers in patients with XP, although acting by different mechanisms for the 2 types of skin cancer.
Heterozygotes
Swift and Chase (1979) found that in 31 families of XP patients, significantly more blood relatives than spouse controls had had nonmelanoma skin cancer. These family data supported the hypothesis that heterozygosity for XP genes may predispose persons to skin cancer, particularly in association with substantial exposure to sunlight, which might overwhelm their DNA repair enzymes.
Some aspect of DNA repair mechanisms is deficient in 4 other inherited diseases: ataxia-telangiectasia (AT; 208900), Fanconi anemia, Bloom syndrome (BLM; 210900), and Cockayne syndrome. Welshimer and Swift (1982) studied families of homozygotes for these disorders to test the hypothesis that heterozygotes may be predisposed to some of the same congenital malformations and developmental disabilities that are common among homozygotes. Among XP relatives, 11 of 1,100 had unexplained mental retardation, whereas only 3 of 1,439 relatives of FA and AT homozygotes showed mental retardation. Four XP relatives and no FA or AT relatives had microcephaly. Idiopathic scoliosis and vertebral anomalies occurred in excess in AT relatives, while genitourinary and distal limb malformations were found in FA families.
Parshad et al. (1990) identified XP heterozygotes by the finding of elevated frequencies of chromatid breaks and gaps after G2 phase x-irradiation of peripheral blood lymphocytes or skin fibroblasts.
Clinical ManagementKraemer et al. (1988) found that 5 patients with XP had a reduction in the number of skin cancers during treatment with isotretinoin: they had a total of 121 tumors in a 2-year interval before treatment and a total of 25 tumors during 2 years of treatment. After the drug was discontinued, the tumor frequency increased at a mean of 8.5-fold over the frequency during treatment. All patients experienced mucocutaneous toxic effects, however, and abnormalities of triglycerides, liver function, or the skeleton developed in some.
Davis et al. (1994) reported 3 patients in whom strenuous preventive measures for UV light avoidance and shielding resulted in a lack of the degree of cutaneous damage usually seen in this disorder.
PathogenesisCleaver (1968) showed that skin fibroblasts from patients with xeroderma pigmentosum had defective or absent DNA repair in response to ultraviolet radiation damage, whereas normal skin fibroblasts could repair this damage by inserting new bases into DNA. Goldstein and Lin (1972) showed that XP-hamster somatic cell hybrids had normal DNA repair and survived ultraviolet irradiation, indicating that the normal hamster cell was able to complement the defect.
Robbins et al. (1974) noted that 4 XP complementation groups, XPA-XPD, had been found to date, indicating that mutation at any one of at least 4 loci cause defective DNA repair resulting in the clinical phenotype. Although there were no consistent clinical correlations, the groups showed different DNA repair rates: group A less than 2% of normal; group B, 3 to 7% of normal; group C, 10 to 25% of normal; and group D, 25 to 55% of normal. By 1975, 5 forms of XP with deficiency in excision repair had been identified by cell complementation (Kraemer et al., 1975; Cleaver, 1975).
Andrews et al. (1978) demonstrated a correlation between the degree of UV sensitivity of cultured fibroblasts, as measured by colony forming ability, and the severity of neurologic manifestations in patients with XP. The authors concluded that DNA repair is required for maintenance of the functional integrity of the nervous system by preventing premature death of neurons. Stefanini et al. (1980) observed a discrepancy in clinical severity and residual enzyme activity in sibs and suggested somatic back-mutation in the milder sib.
Keijzer et al. (1982) observed that cytoplasts from normal human fibroblasts could complement the defect of xeroderma pigmentosum A cells after fusion with UV-irradiated XPA cells. De Jonge et al. (1983) found that microinjection of cell extracts from either human placenta or HeLa cells into XPA cells restored unscheduled DNA synthesis in these cells after UV irradiation and showed normal resistance to UV irradiation. Yamaizumi et al. (1986) found that the half-life of the injected factor was 14 hrs in XPA cells with maximal effects exerted 2 hrs after microinjection. The factors were sensitive to protease but not to RNase and had a molecular weight of about 160 and 90 kD by gel filtration. The factor fractions did not work in other XP groups.
Cleaver (1994) outlined information on the human diseases due to defects in DNA repair, including the mismatch repair system in familial nonpolyposis colorectal cancer (MSH2; 609309), and provided a highly useful block diagram of the steps involved in major branches of nucleotide excision repair. The initial step involves the DNA-binding proteins XPA and XPE; the latter has 2 subunits. Thereafter, 2 branches, transcription dependent and transcription independent, operate in different regions of the genome to change DNA structure by a helicase action for excision. Some gene products appear unique to these branches; i.e., XPC for transcription independent, CSA (609412) and CSB (609413) for transcription dependent, whereas others are involved in both (XPB and XPD). The damaged region is cut out in a 29- to 30-nucleotide fragment by the action of 2 excision nucleases: one is a complex of ERCC1 (126380) and ERCC4 (133520) that can act on single-stranded and duplex-supercoiled DNA; the other, the XPG product, acts on single-stranded DNA. The remaining single-strand template is protected by single-strand binding protein RPA, and a patch is then synthesized by proliferating cell nuclear antigen (PCNA), DNA polymerase delta/epsilon, and ligase. Cleaver (1994) noted that the ERCC4, ERCC11, and XPF proteins may be identical, a statement proven by Sijbers et al. (1996).
Molecular GeneticsMost Japanese patients with XP group A were found by Tanaka et al. (1990) to have a splice site mutation in the XPA gene (611153.0001). In these patients, reduced amount of mRNA of reduced size was found on Northern blot analysis.
In patients with XPA, Satokata et al. (1992) identified homozygous or compound heterozygous mutations in the XPA gene (611153.0002-611153.0006).
Cleaver et al. (1999) reviewed mutations identified in the XPA gene and their population frequencies.
Genotype/Phenotype CorrelationsStates et al. (1998) performed a mutation analysis on XPA cell lines from 19 American and European patients. Most mutations were deletions and splice site mutations, observed previously in other XPA patients in exon 3, intron 3, or exon 4, that resulted in frameshifts within the DNA-binding region. One new mutation was a point mutation within intron 3 causing a new splice acceptor site that may compete with the original splice acceptor site. Mutations in the DNA-binding region of XPA were from patients with the more severe disease often associated with neurologic complications, whereas mutations in the C terminus of the protein, which interacts with the TFIIH (see 189972) transcription factor, were from patients with milder skin disease only. The rarity of naturally occurring missense mutations in the DNA-binding region of XPA suggests that amino acid changes may be sufficiently tolerated such that patients would have mild symptoms and escape detection.
HistoryHaldane (1936) suggested the possibility of partial sex-linked recessive inheritance in XP. El-Hefnawi et al. (1965) presented useful Egyptian pedigrees with XP and suggested linkage with the ABO blood group locus. Bootsma and Keijzer (1979) studied 8 patients from 6 Egyptian XP families. Three were assigned to complementation group A and 5 to group C. Thus, the families reported by El-Hefnawi et al. (1965) likely comprised at least 2 different complementation groups.
Kraemer et al. (1987) reviewed 850 published cases of XP in articles dating from 1874 to 1982. They stated that xeroderma pigmentosum was first described in Vienna by the Hungarian-born professor of dermatology Moriz Kaposi (born Moritz Kohn), in the textbook of dermatology he published in 1870 with his father-in-law, Ferdinand Hebra (Hebra and Kaposi, 1874). They believed that xeroderma pigmentosum with neurologic abnormalities (de Sanctis-Cacchione syndrome) was first reported in 1883 by Albert Neisser of Breslau, Germany (who also discovered the bacterial cause of gonorrhea, the agent named Neisseria).