Malaria, Susceptibility To
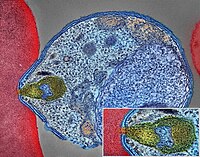
A number sign (#) is used with this entry because variation in several different genes influences susceptibility and resistance to malaria, as well as disease progression and severity. These genes include HBB (141900), ICAM1 (147840), CD36 (173510), CR1 (120620), GYPA (617922), GYPB (617923), GYPC (110750), TNF (191160), NOS2A (163730), TIRAP (606252), FCGR2B (604590), and CISH (602441). In addition, a locus associated with Plasmodium falciparum blood infection level has been mapped to chromosome 5q31-q33 (PFBI; 248310), a locus for susceptibility to mild malaria has been mapped to chromosome 6p21.3 (MALS; 609148), a locus associated with malaria fever episodes has been mapped to chromosome 10p15 (PFFE1; 611384), and a locus for susceptibility to placental malarial infection has been mapped to chromosome 6 (FUT9; 606865). Complete protection from Plasmodium vivax infection is associated with the Duffy blood group-negative phenotype (see 110700). Alpha(+)-thalassemia (141800), the X-linked disorder G6PD deficiency (300908), and Southeast Asian ovalocytosis (166900) are associated with resistance to malaria.
DescriptionMalaria, a major cause of child mortality worldwide, is caused by mosquito-borne hematoprotozoan parasites of the genus Plasmodium. Of the 4 species that infect humans, P. falciparum causes the most severe forms of malaria and is the major cause of death and disease. Although less fatal, P. malariae, P. ovale, and, in particular, P. vivax infections are major causes of morbidity. The parasite cycle involves a first stage in liver cells and a subsequent stage at erythrocytes, when malaria symptoms occur. A wide spectrum of phenotypes are observed, from asymptomatic infection to mild disease, including fever and mild anemia, to severe disease, including cerebral malaria, profound anemia, and respiratory distress. Genetic factors influence the response to infection, as well as disease progression and severity. Malaria is the strongest known selective pressure in the recent history of the human genome, and it is the evolutionary driving force behind sickle-cell disease (603903), thalassemia (see 141800), glucose-6-phosphatase deficiency (300908), and other erythrocyte defects that together constitute the most common mendelian diseases of humans (Kwiatkowski, 2005; Campino et al., 2006).
PathogenesisCompared with other microorganisms, P. falciparum malaria parasites reach very high densities in blood. P. falciparum-infected erythrocytes (PfIRBCs) induce ICAM1 (147840) expression on human brain microvascular endothelial cells (HBMECs), but not on human umbilical vein endothelial cells. PfIRBCs compromise the electrical function of brain endothelium independently of PfIRBC binding phenotype, suggesting a role for soluble parasite factors. By performing genomewide transcriptional profiling of HBMECs after exposure to isogenic PfIRBCs, followed by ELISA for protein identification, Tripathi et al. (2009) identified upregulated molecules involved in immune response, apoptosis and antiapoptosis, inflammatory response, cell-cell signaling, and signal transduction and activation of the NF-kappa-B (see 164011) cascade. Proinflammatory molecules, including CCL20 (601960), CXCL1 (155730), CXCL2 (139110), IL6 (147620), and IL8 (146930), were upregulated more than 100-fold. Tripathi et al. (2009) concluded that PfIRBC exposure to HBMECs results in a predominantly proinflammatory response mediated by NF-kappa-B activation.
By incubating erythrocytes with increasing amounts of anti-CR1 antibodies or soluble CR1 (120620), followed by immunoprecipitation analysis, Tham et al. (2010) showed that the P. falciparum merozoite ligand PfRh4 bound to CR1. Levels of PfRh4 binding correlated with CR1 expression on the erythrocyte surface, which is controlled by the CR1 exon 22 SNP (120620.0001). Binding was reduced in individuals homozygous for low CR1 expression. Parasite invasion of neuraminidase-treated erythrocytes was also reduced. Tham et al. (2010) concluded that CR1 is an erythrocyte receptor used by P. falciparum PfRh4 for sialic acid-independent invasion.
By systematic screening of a library of erythrocyte proteins, Crosnier et al. (2011) identified basigin (BSG; 109480) as a receptor for PfRh5, a P. falciparum ligand essential for blood stage growth of the parasite. Soluble basigin or basigin knockdown inhibited erythrocyte invasion by all P. falciparum strains, and complete blocking was achieved by anti-basigin antibodies. OK(a-) red blood cells, which express the glu92-to-lys (E92K; 109480.0001) variant of basigin, had reduced binding to PfRh5 due to slower association and faster dissociation rates. Another basigin variant, leu90 to pro (L90P), did not interact with PfRh5 at all. Crosnier et al. (2011) concluded that the dependence on a single receptor-ligand pair across many P. falciparum strains may provide novel possibilities for therapeutic intervention.
By screening an array of full-length plasma membrane proteins expressed on human embryonic kidney cells, Turner et al. (2013) identified the endothelial protein C receptor (EPCR; 600646) as a binding partner of domain cassette-8 of the Plasmodium falciparum erythrocyte membrane protein-1 (DC8-PfEMP1). They mapped the PfEMP1 EPCR-binding domain by ELISA with DC8-PfEMP1C8 variants. Further analysis confirmed that PfEmp1 proteins have diverged into CD36 (173510)- and EPCR-binding subtypes. DC8-PfEMP1-expressing and parasitized erythrocytes bound to brain endothelial cells and were inhibited by recombinant EPCR or anti-EPCR antibodies. Turner et al. (2013) proposed that PfEMP1-EPCR-mediated cytoadhesion is the major virulence phenotype for severe malaria.
Cserti-Gazdewich et al. (2012) conducted a prospective analysis of ABO blood groups (616093) and cytoadhesion receptors CD36 and ICAM1 in approximately 2,000 Ugandan children with either uncomplicated or severe malaria, including cerebral malaria (CM), severe anemia (SA), and lactic acidosis (LA). Survival was enhanced in individuals with blood group O and increased monocyte expression of CD36 and ICAM1. Blood group O was nearly 50% in 180,000 adult blood donors and in children with uncomplicated malaria, whereas it was approximately 40% in children with severe malaria. High case fatality rates in cerebral malaria and lactic acidosis were associated with high platelet CD36 expression and thrombocytopenia, whereas severe anemia was characterized by low ICAM1 expression. Logistic regression analysis showed that the odds ratios for the mitigating effects of blood group O, CD36, and ICAM1 phenotypes were greater than that of sickle cell hemoglobin. Cserti-Gazdewich et al. (2012) concluded that selection pressure by P. falciparum continues to shape the human genome.
Using RNA interference-based knockdown of gene expression in CD34 (142230)-positive hematopoietic progenitor cells, induction of ex vivo erythropoiesis, and infection of terminally differentiated erythroblasts with P. falciparum, Egan et al. (2015) identified CD55 (125240) as a critical factor for parasite invasion. CD44 (107269) also appeared to play a role in invasion. Mature erythrocytes from individuals with the Inab phenotype (i.e., CD55 deficiency) of the Cromer blood group system (613793), as well as CD55-knockdown cells, were refractory to invasion, but not to initial attachment, by laboratory and clinical P. falciparum strains. In contrast, the zoonotic P. knowlesi parasite invaded CD55-null and wildtype erythrocytes similarly, suggesting the existence of a P. falciparum-specific ligand for CD55. Egan et al. (2015) proposed that CD55 is an attractive target for malaria therapeutics and suggested that hematopoietic stem cell-based forward screens may be valuable in identifying host determinants of malaria pathogenesis.
Using an antibody array to assess the level of 28 receptors in the livers of 2 substrains of BALB/c mice, Kaushansky et al. (2015) determined that 9 receptors, including EphA2, were present at elevated levels in the substrain that is more susceptible to the murine malaria parasite, Plasmodium yoelii. Infection of a murine hepatocyte line with P. yoelii showed that cells with the highest EphA2 levels also had the highest infection rate. Infection of mice revealed a strong preference for hepatocytes with high EphA2 levels. Infection was inhibited by antibody to the extracellular portion of EphA2 in a dose-dependent manner. EphA2 -/- mice had a lower liver-stage parasite burden and a delay in blood-stage infection compared with wildtype mice. Hepatocytes with high EphA2 levels also showed more effective parasitophorous vacuole membrane development, which is critical for liver-stage development. Further analysis showed that malaria sporozoite invasion involved interaction between EphA2 and parasite 6-cys proteins.
MappingRihet et al. (1998) provided evidence for linkage of the level of blood infection with Plasmodium falciparum and chromosome region 5q31-q33 (see 248310).
Flori et al. (2003) demonstrated linkage of mild malaria to the MHC region in an urban population living in an endemic area in Burkina Faso (see 609148).
Timmann et al. (2007) reported significant association between malaria fever episodes and a locus on chromosome 10p15 (PFFE1; 611384) in a rural Ghanaian population.
Fortin et al. (2002) reviewed the mapping of gene effects in malaria, both in humans and in mice, using population studies and experimental models of malaria susceptibility.
Associations Pending Confirmation
In a genomewide association study of patients with severe malaria and unaffected controls from Ghana, Timmann et al. (2012) identified novel resistance loci for severe malaria within the ATP2B4 gene (108732) on chromosome 1q32.1 and near the MARVELD3 gene (614094) on chromosome 16q22.2. Several SNPs within the ATP2B4 gene showed significant association, with rs10900585 within intron 2 showing strongest association (odds ratio = 0.65; P = 6.1 x 10(-9)). ATP2B4 encodes the major Ca(2+) pump in erythrocytes, the host cells of the pathogenic stage of malaria, and Timmann et al. (2012) hypothesized that variants in ATP2B4 may disturb homeostasis of intraerythrocytic Ca(2+) concentrations and impact parasite reproduction and maturation. The associated SNP on chromosome 16q22.2, 2334880 (odds ratio = 1.24; P = 3.9 x 10(-8)), is located 6.4 kb upstream of the MARVELD3 gene. The MARVELD3 product is part of tight junction structures of epithelial and vascular endothelial cells, and Timmann et al. (2012) noted that endothelial adherence is important in the pathology of severe malaria.
In a multicenter genomewide association study of severe malaria in African children from 9 countries, Malaria Genomic Epidemiology Network (2015) identified a severe malaria resistance locus on chromosome 4 between the FREM3 gene (608946) and a cluster of 3 glycophorin genes (GYPE (138590), GYPB, and GYPA), all of which have a functional role in erythrocyte invasion by P. falciparum.
Molecular GeneticsVariation in HBB and Resistance to Malaria
In a review, Kwiatkowski (2005) noted that 3 coding SNPs in the HBB gene confer resistance to malaria and have risen to high frequency in different populations: HbS (141900.0243), HbC (141900.0038), and HbE (141900.0071). The HbS allele is maintained at a frequency of 10% in malaria-endemic regions, including sub-Saharan Africa and parts of the Middle East. HbS homozygotes have sickle-cell disease (603903), a debilitating and often fatal disorder. The heterozygous state, denoted HbAS, is not associated with any clinical abnormality and confers a 10-fold increase in protection from life-threatening malaria and lesser protection against mild malaria. The HbC allele is found in several parts of West Africa, but is less common than HbS. Homozygotes have relatively mild hemolytic anemia, and both homozygotes and heterozygotes are protected against severe malaria, though homozygotes show substantially greater protection. HbE is common in Southeast Asia. Homozygotes generally have symptomless anemia, and erythrocytes from HbE heterozygotes are resistant to invasion by P. falciparum.
Rihet et al. (2004) surveyed 256 individuals (71 parents and 185 sibs) from 53 families in Burkina Faso over 2 years and found that hemoglobin C carriers were found to have less frequent malaria attacks than AA individuals within the same age group (P = 0.01). Analysis of individual hemoglobin alleles yielded a negative association between Hb C and malaria attack (P = 0.00013). Analyses that took into account confounding factors confirmed the negative association of Hb C with malaria attack (P = 0.0074) and evidenced a negative correlation between Hb C and parasitemia (P = 0.0009).
Fairhurst et al. (2005) reported a marked effect of hemoglobin C on the cell-surface properties of P. falciparum-infected erythrocytes involved in pathogenesis. Relative to parasite-infected normal erythrocytes (Hb AA), parasitized AC and CC erythrocytes showed reduced adhesion to endothelial monolayers expressing CD36 (173510) and intercellular adhesion molecule-1 (ICAM1; 147840). They also showed impaired rosetting interactions with nonparasitized erythrocytes, and reduced agglutination in the presence of pooled sera from malaria-immune adults. Abnormal cell-surface display of the main variable cytoadherence ligand, PfEMP-1 (P. falciparum erythrocyte membrane protein-1), correlated with these findings. The abnormalities in PfEMP-1 display were associated with markers of erythrocyte senescence, and were greater in CC than in AC erythrocytes. Fairhurst et al. (2005) suggested that hemoglobin C might protect against malaria by reducing PfEMP1-mediated adherence of parasitized erythrocytes, thereby mitigating the effects of their sequestration in the microvasculature.
Ayodo et al. (2007) performed an association study combined with evidence of natural selection. The association study tested 10 putative resistance variants in 471 severe malaria cases (mean age 2.6 years) and 474 controls (mean age 16.9 years) from the Luo tribe, who live in a malaria-endemic region of Kenya. The authors replicated associations with HBB and CD36. In the selection study, Ayodo et al. (2007) assembled population control samples from the Masai, Kikuyu, and Yoruba ethnic groups. They found that the same variants are unusually differentiated between the Luo and Yoruba (also historically exposed to malaria in Nigeria) and the Masai and Kikuyu tribes (both living in nonendemic regions of Kenya). Although evidence of association for HBB and CD36 was only moderate by the association analysis alone, formal combination of evidence of association with evidence from the selection test yielded greatly increased significance, up to P = 0.000018 for HBB and P = 0.00043 for CD36. Ayodo et al. (2007) concluded that they empirically demonstrated the theoretical concept of increasing statistical power by orders of magnitude to detect disease variants by combining association analysis with evidence of natural selection.
In a genomewide association study of patients with severe malaria and unaffected controls from Ghana, Timmann et al. (2012) confirmed the protective effect of sickle cell trait.
Thalassemia and Resistance to Malaria
The suggestion that alpha(+)-thalassemia (141800) has achieved a high frequency in some populations as a result of selection by malaria is based on a number of epidemiologic studies. In the southwest Pacific region, there is a striking geographic correlation between the frequency of alpha(+)-thalassemia and the endemicity of Plasmodium falciparum. Allen et al. (1997) undertook a prospective case-control study of children with severe malaria on the north coast of Papua New Guinea, where malaria transmission is intense and alpha(+)-thalassemia affects more than 90% of the population (homozygotes comprise approximately 55% and heterozygotes 37% of the population). Compared with normal children, the risk of having severe malaria was 0.40 in alpha(+)-thalassemia homozygotes and 0.66 in heterozygotes. Unexpectedly, the risk of hospital admission with infections other than malaria also was reduced to a similar degree in homozygotes (0.36) and heterozygotes (0.63). This clinical study demonstrated that a malaria resistance gene protects against disease caused by infections other than malaria. A reduction in mortality greater than that attributable directly to malaria had been observed after the prevention of malaria by insecticides, chemoprophylaxis, and insecticide-impregnated bed nets. Previous observations that direct malaria mortality cannot account for observed hemoglobin S gene frequencies suggest that the findings of this study may apply equally to other malaria resistance genes.
In a study of the epidemiology of childhood malaria on the southwestern Pacific island of Espiritu Santo in Vanuatu, Williams et al. (1996) found that, paradoxically, both the incidence of uncomplicated malaria and the prevalence of splenomegaly, an index of malarial infection, were significantly higher in young children with alpha(+)-thalassemia than in normal children. Furthermore, this effect was most marked in the youngest children and for the nonlethal parasite Plasmodium vivax. The authors speculated that the alpha(+)-thalassemias may have been selected for the ability to increase susceptibility to P. vivax, which, by acting as a natural vaccine in this community, induced limited cross-species protection against subsequent severe P. falciparum malaria.
Variation in FY and Resistance to P. Vivax Infection
The Duffy-null phenotype (see 110700), which results from a promoter SNP in the DARC gene (613665.0002), provides complete protection against P. vivax infection (Kwiatkowski, 2005).
G6PD Deficiency and Resistance to Malaria
Among Nigerian children with convulsions and heavy parasitemia from falciparum malaria, Martin et al. (1979) noted a reduced frequency of G6PD deficiency (305900), an X-linked disorder. They pointed out that the only support for a role of malaria in selecting for deficiency genes had been geographic association. The mechanism of protection of G6PD-deficient cells against falciparum malaria was worked out by Friedman and Trager (1981). G6PD is critical to the regeneration of NADPH, a coenzyme that is essential for protection against and repair of oxidative damage. Red cells deficient in G6PD are more sensitive to hydrogen peroxide generated by the malaria parasite. The loss of potassium from the cell and from the parasite is largely responsible for the death of the parasite. The fava bean contains a variety of substances that increase the red cells' sensitivity to oxidants. Eating fava beans and perhaps other foods as yet not identified would be expected to increase the level of protection against malaria in people who are heterozygous for G6PD deficiency and for thalassemia. Fetal red cells likewise have an increased sensitivity to oxidants and a resulting resistance to malaria. This is true of adult cells that have unusually high concentration of fetal hemoglobin. Roth et al. (1983) found that G6PD-deficient red cells of Sardinian hemizygotes and heterozygotes supported growth of the Plasmodium falciparum parasite in vitro only about one-third as well as normal red cells. No abnormality of growth could be demonstrated in red cells from Sardinians with the beta-zero-thalassemia trait. The authors suggested that the data support a selective advantage of G6PD deficiency in malarious areas; the advantage of the female heterozygote may be particularly strong if resistance to malaria equals that in the hemizygous male, without the risk of fatal hemolysis.
That resistance to severe malaria is the basis of the high frequency of G6PD deficiency and that both hemizygotes and heterozygotes enjoy an advantage was established by Ruwende et al. (1995) in 2 large case-control studies of more than 2,000 African children. They found that the common African form of G6PD deficiency (G6PD A-; 305900.0002) was associated with a 46 to 58% reduction in risk of severe malaria for both female heterozygotes and male hemizygotes. A mathematical model incorporating the measured selective advantage against malaria suggested that a counterbalancing selective disadvantage, associated with this enzyme deficiency, has retarded its rise in frequency in malaria-endemic regions.
Cappadoro et al. (1998) found that with 5 different strains of Plasmodium falciparum, there was no significant difference in either invasion or maturation when the parasites were grown in either normal or G6PD-deficient (Mediterranean variant; 305900.0006) erythrocytes. With all of these strains and at different maturation stages, they were unable to detect any difference in the amount of P. falciparum-specific G6PD mRNA in normal versus deficient parasitized erythrocytes. By contrast, in studies of phagocytosis of parasitized erythrocytes by human adherent monocytes, they found that when the parasites were at the ring stage, deficient ring-stage parasitized erythrocytes (RPE) were phagocytized 2.3 times more intensely than normal RPEs, whereas there was no difference when the parasites were at the more mature trophozoite stage, i.e., trophozoite-stage parasitized erythrocytes (TPEs). The level of reduced glutathione was remarkably lower in deficient RPEs compared with normal RPEs. Cappadoro et al. (1998) concluded that impaired antioxidant defense in deficient RPEs may be responsible for membrane damage followed by phagocytosis. Because RPEs, unlike TPEs, are nontoxic to phagocytes, the increased removal by phagocytosis of RPEs would reduce maturation to TPEs and to schizonts and may be a highly efficient mechanism of malaria resistance in deficient subjects.
Louicharoen et al. (2009) investigated the effect of the G6PD-Mahidol 487A variant (305900.0005) on human survival related to P. vivax and P. falciparum malaria in Southeast Asia. They showed that strong and recent positive selection has targeted the Mahidol variant over the past 1,500 years. The authors found that the G6PD-Mahidol variant reduces vivax, but not falciparum, parasite density in humans, which indicates that P. vivax has been a driving force behind the strong selective advantage conferred by this mutation.
Variation in GYPA and Resistance to Malaria
Red cells with the rare En(a-) variant of GYPA (617922) are resistant to falciparum malaria (Pasvol et al., 1982).
Variation in GYPB and Resistance to Malaria
Red cells with the rare U(-) variant of GYPB (617923) are relatively resistant to invasion by P. falciparum (Pasvol and Wilson, 1982).
Variation in GYPC and Resistance to Malaria
Deletion of exon 3 in the GYPC gene (110750.0002) has been found in Melanesians; this alteration changes the serologic phenotype of the Gerbich (Ge) blood group system (110750), resulting in Ge negativity (Booth and McLoughlin, 1972; Serjeantson et al., 1994). The GYPC exon 3 deletion allele reaches a high frequency (46.5%) in coastal areas of Papua New Guinea where malaria is hyperendemic (Patel et al., 2001). Plasmodium falciparum erythrocyte-binding antigen-140 (EBA140, also known as BAEBL) binds with high affinity to the surface of human erythrocytes. Maier et al. (2003) showed that the receptor for EBA140 is glycophorin C and that this interaction mediates a principal P. falciparum invasion pathway into human erythrocytes. EBA140 does not bind to GYPC in Ge-negative erythrocytes, nor can P. falciparum invade such cells using this invasion pathway. This provides compelling evidence that Ge negativity has arisen in Melanesian populations through natural selection by severe malaria.
Southeast Asian Ovalocytosis and Resistance to Cerebral Malaria
Kidson et al. (1981) found that ovalocytic erythrocytes from Melanesians were resistant to invasion by malaria parasites. Baer (1988) suggested that Malaysian elliptocytosis (166900) may be a balanced polymorphism, i.e., that individuals homozygous for the elliptocytosis allele may be differentially susceptible to mortality, whereas the heterozygote is at an advantage. Hadley et al. (1983) showed that Melanesian elliptocytes were highly resistant to invasion by Plasmodium knowlesi and P. falciparum in vitro.
The band 3 variant in southeast Asian ovalocytosis (109270.0002) may prevent cerebral malaria, but it exacerbates malarial anemia and may also increase acidosis, a major determinant of mortality in malaria. Allen et al. (1999) undertook a case-control study of children admitted to hospital in a malarious area of Papua New Guinea. The 24-bp deletion, detected by PCR, was present in 0 of 68 children with cerebral malaria, compared with 6 (8.8%) of 68 matched community controls. Median hemoglobin levels were 1.2 g/dl lower in malaria cases with southeast Asian ovalocytosis than in controls (P = 0.035), but acidosis was not affected. The band 3 protein mediates the cytoadherence of parasitized erythrocytes in vitro. The remarkable protection that the variant affords against cerebral malaria may offer a valuable approach to a better understanding of the mechanisms of adherence of parasitized erythrocytes to vascular endothelium and the pathogenesis of cerebral malaria.
Variation in CD36 and Susceptibility or Resistance to Cerebral Malaria
CD36 is a major receptor for Plasmodium falciparum-infected erythrocytes. Aitman et al. (2000) found that African populations contain an exceptionally high frequency of mutations in CD36 (173510). Unexpectedly, these mutations (173510.0002 and 173510.0003) that cause CD36 deficiency (608404) were associated with susceptibility to severe cerebral malaria, suggesting that the presence of distinct CD36 mutations in Africans and Asians is due to some selection pressure other than malaria.
In 475 adult Thai patients with P. falciparum malaria, Omi et al. (2003) screened for variation in the CD36 gene and examined possible association between CD36 polymorphisms and the severity of malaria. They identified 9 CD36 polymorphisms with a frequency of more than 15% for the minor allele. Of these, the -14T-C allele in the upstream promoter region and the -53G-T allele in the downstream promoter region were significantly decreased in patients with cerebral malaria compared with those with mild malaria. Linkage disequilibrium (LD) analysis between the 9 common polymorphisms revealed 2 blocks with strong LD in the CD36 gene; the -14T-C and -53G-T polymorphisms were within the upstream block of 35 kb from the upstream promoter to exon 8. Another polymorphism, consisting of 12 TG repeats in intron 3 (173510.0004), was strongly associated with reduction in the risk of cerebral malaria. Omi et al. (2003) demonstrated by RT-PCR amplification that this IVS3(TG)12 polymorphism is involved in the nonproduction of the variant CD36 transcript that lacks exons 4 and 5. Because exon 5 of the gene is known to encode the ligand-binding domain for P. falciparum-infected erythrocytes, IVS3(TG)12 itself or a primary variant on the haplotype with IVS3(TG)12 may be responsible for protection from cerebral malaria in Thailand.
Ayodo et al. (2007) sought to demonstrate that statistical power to detect disease variants can be increased by weighting candidates by their evidence of natural selection. Although evidence of association for HBB and CD36 was only moderate by an association analysis alone, formal combination of evidence of association with evidence from a selection test yielded greatly increased significance, up to P = 0.000018 for HBB and P = 0.00043 for CD36.
Variation in CR1 and Resistance to Malaria
The Knops blood group system (607486) is a system of antigens located on CR1. Rowe et al. (1997) demonstrated that CR1 is involved in malarial rosetting, a process associated with cerebral malaria, which is the major cause of mortality in Plasmodium falciparum malaria. They showed that rosette formation was considerably reduced with Sl(a-) Knops phenotype RBCs, indicating that this antigen on CR1 is involved in rosetting. Because Sl(a-) is more common in persons of African ancestry, a protective role was suggested (Moulds and Moulds, 2000).
CR1-deficient RBCs show greatly reduced rosetting, leading Cockburn et al. (2004) to hypothesize that if rosetting is a direct cause of malaria pathology, CR1-deficient individuals should be protected against severe disease. They showed that RBC CR1 deficiency occurs in up to 80% of healthy individuals from the malaria-endemic regions of Papua New Guinea. This RBC CR1 deficiency is associated with polymorphisms in the CR1 gene (e.g., 120620.0001) and, unexpectedly, with alpha-thalassemia, a common genetic disorder in Melanesian populations. Analysis of a case-control study demonstrated that the CR1 polymorphisms and alpha-thalassemia independently confer protection against severe malaria. Thus, Cockburn et al. (2004) identified CR1 as a new malaria resistance gene and provided compelling evidence that rosetting is an important parasite virulence phenotype that should be a target for drug and vaccine development.
Variation in ICAM1 and Susceptibility to Cerebral Malaria
The malarial parasite Plasmodium falciparum has acted as a potent selective force on the human genome. The particular virulence of this organism was thought to be due to the adherence of parasitized red blood cells to small vessel endothelium through several receptors, including CD36, thrombospondin (THBS1; 188060), and ICAM1, and parasite isolates differ in their ability to bind to each. Immunohistochemical studies implicated ICAM1 as having potential importance in the pathogenesis of cerebral malaria, leading Fernandez-Reyes et al. (1997) to reason that if any single receptor were involved in the development of cerebral malaria, then in view of the high mortality of that complication, natural selection should have produced variants with reduced binding capacity. Fernandez-Reyes et al. (1997) amplified and sequenced the N-terminal immunoglobulin-like domain of the ICAM1 gene from the genomic DNA of 24 asymptomatic children in Kilifi, Kenya. The only mutation found was an A-to-T transversion at nucleotide 179, causing a lys29-to-met substitution (K29M; 147840.0001), which the authors called 'ICAM1 Kilifi.' In studies of the association of the K29M polymorphism with cerebral malaria, they found, to their surprise, that the homozygous ICAM1 Kilifi genotype was associated with susceptibility to cerebral malaria with a relative risk of 2.23, and heterozygotes with a relative risk of 1.39. The frequency of the K29 allele was 0.668 and the frequency of the M29 Kilifi allele was 0.332. Fernandez-Reyes et al. (1997) noted that, while this association strengthened the link between ICAM1 and cerebral malaria, a mutation that confers susceptibility is unlikely to have arisen at such high frequency in the absence of some counteractive selective advantage. These counterintuitive results had implications for the mechanism of malaria pathogenesis, resistance to other infectious agents, and transplant immunology. The Kilifi allele was not identified in 99 unrelated Caucasians or in 40 multigeneration families from the CEPH collection. Screening of 20 Gambian samples produced a similar frequency of the Kilifi allele to that seen in Kenya.
Bellamy et al. (1998) found no association between the ICAM1 Kilifi variant and cerebral malaria in a case-control study of West Africans.
Variation in Major Histocompatibility Complex Genes and Resistance to Severe Malaria
By means of a large case-controlled study of malaria in West African children, Hill et al. (1991) showed that HLA-Bw53 (see HLA-B; 142830) and the HLA class II haplotype, DRB1*1302/DQB1*0501 (see HLA-DRB1; 142857), were independently associated with protection from severe malaria. The antigens listed are common in West Africans but rare in other racial groups. In this population, they account for as great a reduction in disease incidence as the sickle-cell hemoglobin variant. Although the relative strength of the protection is less than that of the sickle-cell variant, the greater frequency of the DQB1 (see HLA-DQB1; 604305) polymorphism makes the net effect on resistance to malaria comparable. The findings support the hypothesis that the extraordinary polymorphism of major histocompatibility complex genes has evolved primarily through natural selection by infectious pathogens.
Hill et al. (1992) further investigated the protective association between HLA-B53 and severe malaria by sequencing peptides eluted from this molecule followed by screening of candidate epitopes from pre-erythrocytic-stage antigens of Plasmodium falciparum in biochemical and cellular assays. Among malaria-immune Africans, they found that HLA-B53-restricted cytotoxic T lymphocytes recognized a conserved nonamer peptide from liver-stage-specific antigen-1 (LSA-1), but no HLA-B53-restricted epitopes were identified in other malaria antigens. The findings of this 'reverse immunogenetic' approach indicated a possible molecular basis for this HLA-disease association and supported the candidacy of LSA-1 as a component for a malaria vaccine.
Sjoberg et al. (1992) found that levels of antibody to a major malarial antigen developing in individuals living in northern Liberia, where malaria is holoendemic and perennial, were more concordant within monozygotic twin pairs than in dizygotic pairs or in age- and sex-matched sibs living under similar environmental conditions. The results supported the conclusion that the antibody responses were genetically regulated. No association was found with different HLA class II alleles and haplotypes, suggesting that the variation in the antibody response found in this study reflected the impact of factors encoded by genes outside the HLA class II region.
Variation in TNF and Susceptibility to Cerebral Malaria
Because fatal cerebral malaria is associated with high circulating levels of TNFA (TNF; 191160), McGuire et al. (1994) undertook a large case-control study in Gambian children. The study showed that homozygotes for the TNF2 allele (-308G-A; 191160.0004), a variant of the TNFA gene promoter region, had a relative risk of 7 for death or severe neurologic sequelae due to cerebral malaria. Although the TNF2 allele is in linkage disequilibrium with several neighboring HLA alleles, McGuire et al. (1994) showed that this disease association was independent of HLA class I and class II variation. The data suggested that regulatory polymorphisms of cytokine genes can affect the outcome of severe infection. The maintenance of the TNF2 allele at a gene frequency of 0.16 in The Gambia implies that the increased risk of cerebral malaria in homozygotes is counterbalanced by some biologic advantage.
Through systematic DNA fingerprinting of the TNF promoter region, Knight et al. (1999) identified a SNP (-376G-A; 191160.0003) that caused the helix-turn-helix transcription factor OCT1 (POU2F1; 164175) to bind to a novel region of complex protein-DNA interactions and alter gene expression in human monocytes. The OCT1-binding genotype, found in approximately 5% of Africans, was associated with 4-fold increased susceptibility to cerebral malaria in large studies comparing cases and controls in West African and East African populations, after correction for other known TNF polymorphisms and linked HLA alleles.
Variation in NOS2A and Resistance to Malaria
Kun et al. (1998) examined whether high plasma concentrations of nitric oxide found in severe malaria were due to variation in the promoter region of NOS2 (163730). Heterozygosity for a -969G-C SNP (163730.0002) was present in 30 of 100 Gambian children with mild malaria, but in only 17 of 100 Gambian children with severe malaria. The SNP was not found in any of 100 Germans. Heterozygous individuals were also at a significantly lower risk of reinfection.
From studies in Tanzania and Kenya, Hobbs et al. (2002) identified a novel SNP, -1173C-T (163730.0001), in the NOS2A promoter that was significantly associated with protection from symptomatic malaria and severe malarial anemia.
Variation in TIRAP and Resistance to Malaria
Khor et al. (2007) reported a case-control study of 6,106 individuals from the U.K., Vietnam, and several African countries with invasive pneumococcal disease (see 610799), bacteremia, malaria, and tuberculosis (607948). Genotyping 33 SNPs, they found that heterozygous carriage of a leucine substitution of ser180 (606252.0001) in TIRAP (606252) was associated independently with all 4 infectious diseases in the different study populations. Combining the study groups, they found substantial support for protective effect of S180L heterozygosity against these infectious diseases.
Variation in FCGR2B and Resistance to Malaria
Clatworthy et al. (2007) found an increased frequency of the I232T polymorphism (604590.0001) of the FCGR2B gene (604590) in Asian and African populations, broadly corresponding to regions where malaria is endemic. The systemic lupus erythematosus (SLE; 152700)-associated I232T polymorphism was associated with enhanced phagocytosis of Plasmodium falciparum-infected human erythrocytes. Clatworthy et al. (2007) concluded that FCGR2B is important in controlling the immune response to malaria parasites and suggested that polymorphisms predisposing to SLE in Asians and Africans may be maintained because the variants reduce susceptibility to malaria.
By comparing genotypes of patients with SLE from Hong Kong and the UK with those of ethnically matched controls, followed by metaanalysis using with other studies on southeast Asian and Caucasian SLE patients, Willcocks et al. (2010) found that homozygosity for T232 of the I232T polymorphism was strongly associated with SLE in both ethnic groups. When studies in Caucasians and southeast Asians were combined, T232 homozygosity was associated with SLE with an odds ratio of 1.73 (P = 8.0 x 10(-6)). Willcocks et al. (2010) noted that the T232 allele of the SNP is more common in southeast Asians and Africans, populations where malaria is endemic, than in Caucasians. Homozygosity for T232 was significantly associated with protection from severe malaria in Kenyan children (odds ratio = 0.56; P = 7.1 x 10(-5)), but no association was found with susceptibility to bacterial infection. Willcocks et al. (2010) proposed that malaria may have driven retention of a polymorphism predisposing to a polygenic autoimmune disease and thus may begin to explain the ethnic differences seen in the frequency of SLE.
Blood Group O and Resistance to Severe Malaria
Rowe et al. (2007) noted that Plasmodium falciparum-induced rosetting (i.e., the spontaneous binding of infected erythrocytes to uninfected erythrocytes) is thought to contribute to the pathogenesis of severe malaria by obstructing microvascular blood flow. Rosetting is reduced in blood group O (see 110300) erythrocytes compared with non-O blood groups, presumably due to group O individuals having disaccharide H antigens resulting from a lack of the terminal glycosyltransferases necessary to produce the trisaccharides found with A and B antigens. Rosettes that do form in group O red cells are smaller and more easily disrupted than those in group A, B, or AB red cells. Rowe et al. (2007) confirmed that rosetting was reduced in individuals with blood group O, intermediate in blood groups A and B, and highest in group AB. A matched case control study of 567 Malian children found that group O was present in only 21% of severe malaria cases compared with approximately 44% of uncomplicated malaria control cases and healthy controls. Rowe et al. (2007) concluded that group O is associated with a 66% reduction in the odds of developing severe malaria compared with non-O blood groups, and they reported preliminary evidence that similar protection is found in Kenyan children. The authors also proposed that group O does not occur at higher frequency in some malaria endemic regions due to increased susceptibility to cholera and other diarrheal diseases, resulting in balanced polymorphism.
In a genomewide association study of patients with severe malaria and unaffected controls from Ghana, Timmann et al. (2012) confirmed the protective effect of blood group O.
Variation in GNAS and Susceptibility to Severe Malaria
Using metaanalysis combining data from case control and family studies in Gambia, Kenya, and Malawi and a case control study from Ghana, Auburn et al. (2008) detected associations between intronic or conservative SNPs of GNAS (139320) and severe malaria. SNPs with significant associations clustered in the 5-prime end of GNAS. Auburn et al. (2008) proposed that the impact of GNAS on malaria parasite invasion efficacy may alter susceptibility to disease.
Variation in TIM1 and Resistance to Cerebral Malaria
By screening for polymorphisms of TIM1 (HAVCR1; 606518), TIM3 (HAVCR2; 606652), and TIM4 (TIM4D; 610096) in 478 Thai patients infected with Plasmodium falciparum, Nuchnoi et al. (2008) identified a statistically significant association between protection against cerebral malaria and a TIM1 promoter haplotype consisting of 3 derived alleles, -1637G-A (rs7702919), -1549G-C (rs41297577), and -1454G-A (rs41297579). Allele-specific transcription quantification analysis revealed that TIM1 mRNA levels were higher for the protective promoter haplotype than for the other promoter haplotype. Nuchnoi et al. (2008) proposed that engagement of TIM1 and T-cell receptor stimulation may induce antiinflammatory Th2 cytokine production and protect from development of cerebral malaria by downregulating inflammatory cytokines such as TNF (191160) and IFNG (147570).
Variation in IL12B and Susceptibility to Cerebral Malaria
Using a family-based association study with 240 Malian families, Marquet et al. (2008) investigated 21 markers in IL12-related genes for involvement in susceptibility to cerebral malaria (CM). They found that the IL12B (161561) promoter polymorphism rs17860508, in which GC is replaced with CTCTAA, was associated with susceptibility to CM. The CTCTAA allele and the GC/CTCTAA heterozygous genotype were associated with increased risk of CM (P of 0.0002 and 0.00002, respectively). Children with the GC/CTCTAA genotype had a higher risk of CM than children homozygous for either allele (odds ratio of 2.11; P less than 0.0001). Among 134 CM children with a heterozygous parent, a significant number received the CTCTAA allele. Marquet et al. (2008) noted that heterozygosity for rs17860508 is associated with reduced IL12B expression and reduced IL12 secretion, and that low IL12 and IFNG (147570) levels are associated with CM. They proposed that Th1 responses may reduce the parasite load and severe malaria risk.
Variation in FUT9 and Susceptibility to Placental Malaria Infection
Sikora et al. (2009) carried out a nested case-control study on 180 Mozambican pregnant women with placental malaria infection and 180 controls within an intervention trial of malaria prevention. Subjects were genotyped at 880 SNPs in a set of 64 functionally related genes involved in glycosylation and innate immunity. A T-C SNP (rs3811070) located in the 5-prime untranslated region (UTR) of the FUT9 gene (606865) on chromosome 6q16 was significantly associated with placental malaria infection (odds ratio, 2.31; corrected p = 0.038). Haplotype analysis revealed a similarly strong association for a common 4-SNP TTCA haplotype including rs3811070. The TTCA haplotype spans 40 kb in the 5-prime UTR and contains the second exon of FUT9. The FUT9 gene encodes a fucosyltransferase that catalyzes the last step in the biosynthesis of the Lewis-x antigen, which forms part of the Lewis blood group-related antigens. Sikora et al. (2009) suggested an involvement of this antigen in the pathogenesis of placental malaria infection.
Variation in FCGR2A and Susceptibility to Severe Malaria
The his131-to-arg (H131R; 146790.0001) polymorphism in the extracellular domain of FCGR2A reduces the receptor's affinity for IgG2 and IgG3 isotypes (see 147100) but increases its binding of C-reactive protein (CRP; 123260). By studying 2,504 Ghanaian children with severe malaria and 2,027 healthy matched controls, Schuldt et al. (2010) found that homozygosity for 131R was positively associated with severe malaria (odds ratio = 1.20; p = 0.007; p corrected for multiple testing = 0.021), and, after stratification for phenotypes, with severe anemia (odds ratio = 1.33; p = 0.001; p corrected = 0.009), but not with cerebral malaria or other malaria complications or with parasitemia levels. Schuldt et al. (2010) concluded that the CRP-binding variant of FCGR2A is associated with malarial anemia, suggesting a role for CRP defense mechanisms in pathogenesis of this condition.
Variation in PIEZO1 and Resistance to Malaria
Ma et al. (2018) identified a novel PIEZO1 allele, E756del (611184.0016), that is present in one-third of populations of African descent and showed that it causes red blood cell (RBC) dehydration and attenuates Plasmodium infection. Ma et al. (2018) acquired blood samples from 25 healthy African American donors negative for mutations resulting in hemoglobinopathies or alpha-thalassemia and found that 9 (36%) were heterozygous for the E756del allele. Ma et al. (2018) infected RBCs from E756del carriers and controls with P. falciparum in vitro and found that parasitemia was significantly lower for E756del carriers.
Variation in SLC40A1 and Resistance to Malaria
Zhang et al. (2018) hypothesized that mutations in FPN1 (SLC40A1; 604653) that increase ferroportin (FPN) levels would protect humans from malaria infection and be evolutionarily enriched in malaria-endemic regions. They found that the FPN gln248-to-his variant (Q248H; rs11568350) occurs in sub-Saharan African populations with a heterozygote prevalence of 2.2 to 20%, depending on location. The mutation renders FPN resistant to hepcidin-induced degradation, and carriers have lower hemoglobin concentrations than controls, consistent with the hypothesis that high FPN levels in erythroblasts export iron and diminish hemoglobin production. Zhang et al. (2018) found 3 heterozygotes for Q248H among 27 African Americans. Immunoblot analyses confirmed that FPN levels were increased in the red blood cells of Q248H heterozygotes relative to control donors, indicating that the mutation could have health consequences in carriers of African descent. In a study of 66 hospitalized Zambian children less than 6 years of age with uncomplicated P. falciparum malaria, Zhang et al. (2018) observed the Q248H mutation in 19.7% (12 heterozygotes, 1 homozygote). Compared to patients with a wildtype allele, who had a median of 189,667 parasites/microliter, Q248H patients had 28,000 parasites/microliter (2-sided Fisher's test and chi-square test, p = 0.025). Q248H patients also experienced less fulminant malaria, manifested by tolerance of longer fever times before presentation to the hospital (median of 69 versus 37 hours, Q248H versus wildtype, p = 0.1). Zhang et al. (2018) also investigated the effects of Q248H mutation on malarial infection in 290 primiparous Ghanaian women. Primiparae are particularly prone to placental malaria, because acquired immunity against parasites adhering to the placental syncytiotrophoblast is insufficiently developed in the first pregnancy. Of 290 women, 8.6% were Q248H carriers (24 heterozygotes, 1 homozygote). Present or past placental P. falciparum infection occurred less frequently in Q248H carriers (44.0%) than in women with the respective wildtype allele (70.2%, p = 0.007), even after adjusting by logistic regression for known predictors